If it is possible to tap in to earth’s telluric currents to generate an electrical charge, is it possible to do something similar with water? River and sea water are constantly moving after all. According to Robert Murray Smith, an earth battery relies on the earth’s dampness to act as an electrolyte, conveying current. Water, whether from a river, sea or tap, also acts as an electrolyte.
What are electrolytes?
An electrolyte is a medium containing ions. Ions from salts or acids can be dissolved in to a polar solvent such as water. The ion-containing salt or acid splits in to cations (positive) and anions (negative) when dissolved in the solvent. When an electrical charge is applied to the solution, the negatively charged anions are attracted to the positively charged anode, and the positively charged cations are attracted to the negatively charged cathode - opposites attract. In this way the movement of the cations and anions through the solution to each electrode, creates an electrical current. All good so far. What Robert Murray-Smith demonstrated with the earth battery experiment, was that the dampness in the soil acted as the electrolyte. What he didn’t do was apply an external power source like a battery, in order to get a reading from his meter. The external power source in this experiment came from the electrical potential of the Telluric current. So how is this possible?
This table goes through the different solubility properties of ionic compounds and transition metals. Transition metals are metals that contain valence ‘electrons that can participate in the formation of chemical bonds’. Valence electrons are arranged around the outermost shell predominantly of metal atoms. There can only be up to 8 electrons in this outer shell. The number contained in the outer shell gives us some idea of the electric potential of a metal. These electrons are easily knocked off the outer shell and can therefore travel creating current as they move to the more positively charged anode. Here is a great video on how to remember what anodes, anions, cathodes and cations are in terms of their role in electric potential. Galvanic cells and electrolytic cells are different from each other, but so we don’t confuse everyone (and ourselves) just watch this video.
Perpetual water batteries
Developing the electrolyte and electrode theory further, Robert Murray Smith has made a video on his recreation of William Clarke’s Patent no 4153757 for a perpetual water battery in 1979 shows another method of creating current through electrolysis of water flow. It proves that the movement of water around electrodes can create a current. This does not even need to be a raging torrent, as you might see in a large dam or reservoir. It could simply be as much as tapping in to the energy from a river, upstream to downstream. In this patent we see Clarke tapping in to the current from an ordinary tap.
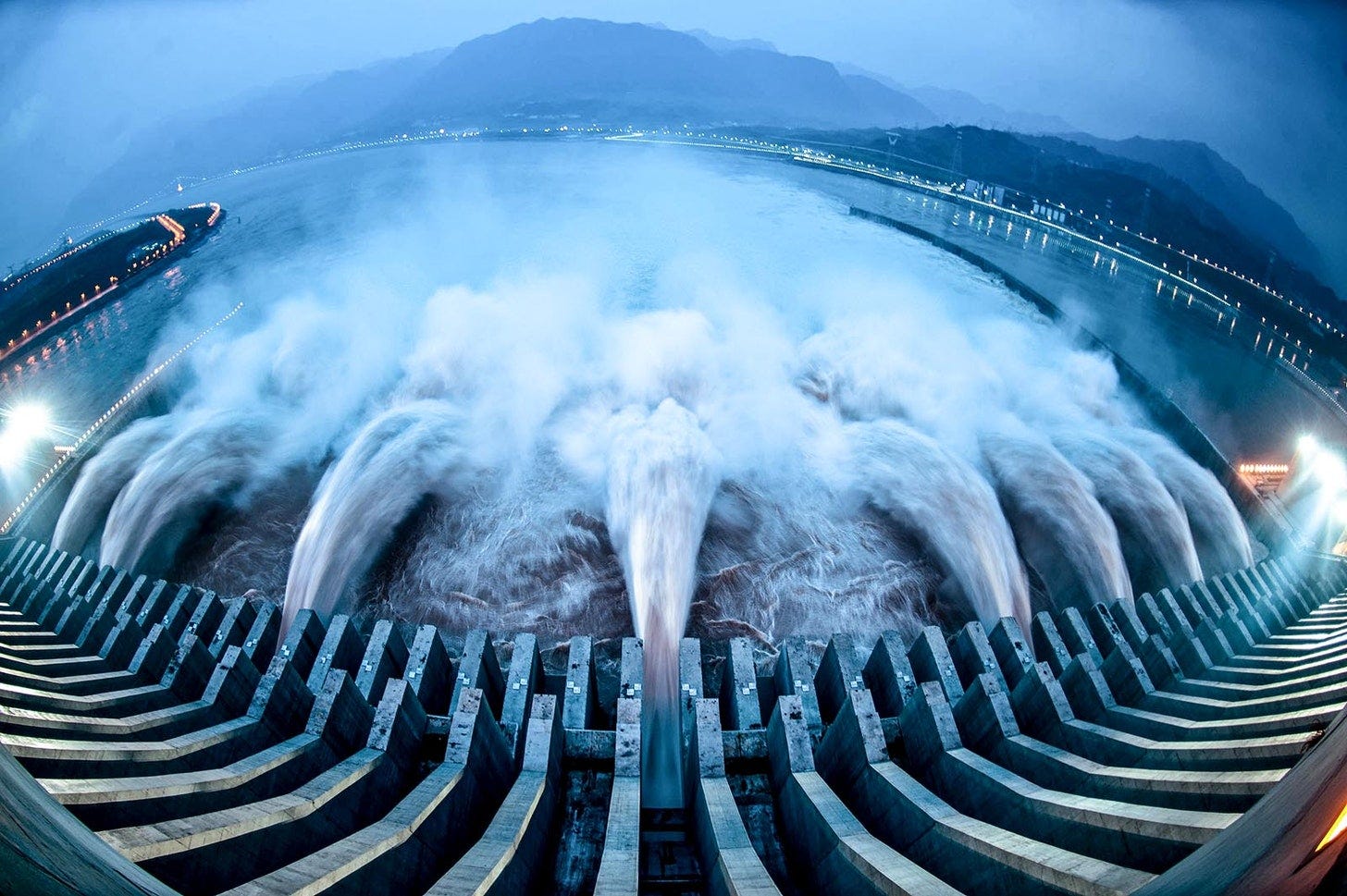
In order to recreate Clarke’s invention, Murray Smith starts with lengths of aluminium and brass tube. With these he sets about making a series of anode and cathode pairs. Each pair is connected to a plastic hinge with a flexible joint so they are able to move in relation to each other in the medium. Each electrode is connected via a conductor to a meter. To test one pair he puts it in water on a heating plate, and then tests a pair just by moving them in relation to each other (waggling) in the water.
Again, as with the experiment with the earth battery, he produces a very small amount of current flow, in this case only 11 microamps. Amperage, as we discussed before is the speed with which the current travels through the conductor measured in units at any given cross sectional point … so in this experiment not very fast. Voltage is the pressure with which the current runs through the wire. The wattage is a combination of the voltage multiplied by the amperage. Here is a great dummies guide on getting to grips with voltage, amperage and wattage.
The cathode, in this case aluminium, which holds a negative charge in relation to brass, is placed upstream, the positively charged anode made of brass, is placed downstream. The flow of water from the positively to negatively charged electrode creates the electrical charge, which is then measured. This video on electroplating explains how the electrons move from a positively charged metal to a negatively charged metal. The solution of water in the stream provide the anions and cations required, to provide enough movement of electrons to create electrical charge.
Cations and anions
Seawater, in contrast to river water, contains an abundance of ions, both cations and anions. Sodium and chlorine make up approximately 86% of all salts in ocean water. The total percentage of salts is approximately 34mg/l, sodium chloride making up 29mg/l. When in solution, sodium chloride is dissolved in to cations of Sodium (Na+) and anions of Chlorine (Cl-). As their polarity is opposite, minus one electron and plus one electron, the two are attracted to each other. Sodium and chlorine concentrations are 333 times higher in seawater than in river water.
Given the abundance of these two ions in seawater, and it’s capacity as an electrical conductor as demonstrated in this video below, we could assume seawater would create a bigger voltage and amperage. Robert Murray Smith interestingly uses carbon electrodes for his seashore experiment so we are not able to compare adequately.
In this other experiment showing how salt water can be used to power a small LED battery with a layer of aluminium and a layer of copper, we also see the significant electric potential of saline or seawater.
Negative energy yields
Hydroelectric power is created by the downward flow of water which turns turbines to create energy. The largest hydroelectric dams in the UK are in Scotland and Wales. The Dinorwig Power Station in Snowdonia uses a pumped-storage system, in that it has two reservoirs of water. The lower reservoir is pumped to the top reservoir using electricity during off peak hours, then descends again using gravity to create power during peak hours. Bizarrely, according to the Wikipedia review on this system, ‘the losses of the pumping process make the plant a net consumer of energy overall, the system increases revenue by selling more electricity during periods of peak demand, when electricity prices are highest.’ In summary, the hydroelectric plant consumes more energy than it produces, but makes up for that loss by charging more for peak hours energy. Of the other four, Ben Cruachan Power Station is also pumped, as is Ffestiniog Power Station, Foyers Power Station however Sloy Power Station takes water from Loch Sloy. There is a natural fall from the Loch to the power station, and the Loch seems to be fed from Glengyle water.
Tapping in to water flows
If the hydroelectric power stations were fitted with large electrodes, along the same design as the experiment run by Murray Smith, as well as turbines, might it be possible to create significantly more power as the water cascades down through the vertical intake to the lower reservoir or outlet? In addition, if the same were applied to the shaft used to pump the water up to the top of the reservoir in the pump models, it might be possible to reclaim some of the expended water return energy. Both of these solutions might be able to tip the hydroelectric dam in to positive energy production, whilst continuing to produce electricity at peak time and accruing the profits from that.
In theory, the same electrode system of current generation could be scaled up and applied in any flow of water, from river source to river mouth, from clean water reservoirs that feed water to water treatment plants, from water treatment plants to homes, drainage and irrigation ditches, water board controlled waterways, pumping stations, canals with locks and weirs, even anti flood systems like the Thames Barrier. Boston in Lincolnshire has recently also had a flood barrier installed following catastrophic flooding in December 2013. It seems foolish not to combine electricity creating technologies with these natural water flows and flood defenses. The same could be applied in inlets and outlets of water to the power stations we discuss further on.
Electrical conductivity of seawater
According to this paper, conductivity of fresh water ranges from 100-2000 umhos per cm. Umho is micromho or the measurement of conductivity in MicroSiemens. The opposite of conductivity is resistivity, or ohm. The more ions a solution contains, the less resistance it has and the more conductive it is. The fewer ions it has the more resistance to electrical current flow. The etymology of current originated in the 14th century, with stem in the French ‘Corant’, meaning ‘that which flows’ or runs. The term ‘current’ was adopted in the 1700’s regarding the flow of electrical force.
The measure of conductivity of seawater is approximately 56,000 MicroSiemens per cm. As we saw from this Stanford paper seawater has nearly double the negative ions to positive ions. Fresh water has very low but fairly equal amounts of negative and positive ions. If we take the upper end of the fresh water range, that means seawater is a minimum of 28 times more conductive than fresh water. This research paper also from Stanford shows that the difference between the electric potential of freshwater and saline water could be used to power 100,000 homes with a flow rate over electrodes of 50 cubic metres per second. The electrode tank or battery is filled with river water (or even gray water), charged, then exchanged for salt water, which produces significantly more electrical potential in discharge. This method could be applied to all areas where river water meets seawater in estuaries and river mouths.
A design by a British engineer RE Pattle in 1954 looked at harnessing seawater and river water to create electricity. The invention proposed the use of a permeable membrane, similar to those found in plants, and using osmotic power to drive the turbines. Different concentrations of salty liquid (seawater/river water) would fill chambers either side of the membrane. The membrane would allow water to pass through to the more salty side, in turn increasing the pressure in that chamber, the pressure for which would be used to power turbines.
What is perplexing, however, is if seawater is so much more conductive than freshwater, why would it not be possible to harness it’s power by running river water through electrode tanks further upstream and sea water through electrode tanks further downstream as per the experiment from Stanford? Perhaps some of that flow power could be used to charge these combined fresh and sea water batteries up, which would function as power storage units when river flow was low and/or the tide was out.
Sea water technologies
Tidal power is similar in concept to that produced by a hydroelectric dam, turning turbines to produce electricity. Tides are reliable! Globally the potential power from tidal systems is only 800 terrawatt hours per year. Wave energy potential runs from 8000-80000 terrawatt hours according to the Tidal Power website but would require significant surface water structures. Thermal energy from the sea could produce up to 10,000 terrawatt hours, osmotic power 2,000 and marine currents, using turbine derived energy, approximately 50,000. These systems would aim to harness power through kinetic and thermal energy, not through electrode based generation.
More recent proposals for the revival of 200 year old ideas on H2 production for energy seem to take the electrode/water theory one step further, with a variety of designs. H2, or hydrogen gas, can be used to create electrical energy in fuel cells and can also replace combustion products in a much more sustainable way. The big issue with this system is that energy is required to create the electrolysis to produce the H2. Similarly to the gas fed power stations and hydroelectric pump based power stations, it seems a complicated and slightly counter-intuitive way of producing energy. But spend money to make money is often considered a capitalist norm.
Burn gas, deionise water, make steam, make electricity
It makes a lot of sense to harness the natural power of the many estuaries and river mouths around the UK. Many of our existing, and some now demolished, power stations are situated along these estuaries, making use of the seawater for cooling. Many of these estuaries are also dotted with electricity substations, ready to distribute electricity. There is a very large one on the River Thames Estuary near to the Grain Tower Battery and Forts. It sits next to the Grain CHP Power Station run by Uniper.
The Grain power station creates electricity from burning gas to heat water to drive steam turbines to create electrical current. The facility is cooled using seawater. Seawater is deionised before it is passed through the boiler for gas combustion to heat, which then creates steam. The presentation linked above highlights that the use of seawater in cooling increases corrosion and deposits in the pipes.
Other sites owned by Uniper that generate electricity by burning gas are the Thames Estuary (seawater), Cottam Development centre on the River Trent (river water), Connah’s Key in Deeside Estuary (seawater), Enfield (Reservoir from River Lee), Taylor’s Lane, London (Grand Union Canal) and Killinghome in Humberside (estuary). It is unclear if all of these sites rely on water cooling, but the potential sources of water are included. Google earth shows that some of the Grain site has been demolished possibly to make way for new initiatives looking at hydrogen power and Blue energy. Blue energy is a system developed along the osmotic energy technology invented by Pattle.
Preventing electrode corrosion
In the Earth Battery video from the last article, we know that metals exposed to water and damp earth are prone to oxidisation or galvanic corrosion. It is galvanic corrosion which allows the copper to dissolve and adhere to the brass as we saw in the electroplating video above. This process is the corrosion of one metal in the presence of another dissimilar metal, where they are in electrical contact through an electrolyte. The metal more prone to oxidisation would corrode.
If we were choosing electrodes for a small or scaled up version of Clarke’s/Murray Smith’s experiment, the pairing of suitable metals would be key, in order to prevent the corrosion of either electrode. This article details the metal pairings that have the least galvanic potential. The green range are the most compatible pairings. Iron, Cadmium, Nickel, Tin and lead seem to be the least corrosive pairings. When creating electrical current we would also need to look at conductivity. Silver, copper, gold, zinc, nickel and platinum being the most conductive metals. Here is a summary of the properties considered when choosing electrodes.
It might also be possible to use compounded metals to create corrosion resistant electrodes particularly for seawater use. Fresh water is many times less corrosive than saltwater. Aluminium Bronze compounds are used in maritime vessels and structures for anti-corrosion properties. The compound can be formed out of a small amount of Aluminium and the remainder of copper. This company, Smith Metal, specialises in these types of metals. There are a variety of percentages of metal blends according to requirement. As aluminium and copper are very conductive metals, it might be possible to create two electrodes with different percentages of aluminium and copper, in order that their anode to cathode conductivity form a good electrical charge. Similarly to the earth battery, protective coatings could also be applied to electrodes, such as graphite.
Nuclear energy
One of the most interesting energy generation systems that uses water is that of the nuclear power plant.
In the pressurised water reactor, Uranium rods are contained in a steel pressure vessel, in to which neutron absorbing rods can be raised and lowered. Cool feedwater is pumped inbetween the uranium rods, which heats the water. It then moves past a pressuriser and is funneled through a heating element in to the steam generator. Steam is formed in the generator in a water tank separate from the feedwater. The water is heated by the elements to produce steam which drives the turbines. The water heated by the uranium rods, looses its heat in the steam generator and is returned as cooled water to start the cycle again. Condensate from the steam generator is funneled out of the turbine system to either cooling lakes or towers.
In the boiling water reactor the feedwater that passes through the uranium rods, is the same water that creates the steam to run the turbine. It still loops round in a closed system back to the reactor vessel, once it is condensed and cooled by running through the condenser tank (which also has a loop of cold water pumped in from cooling lakes or towers).
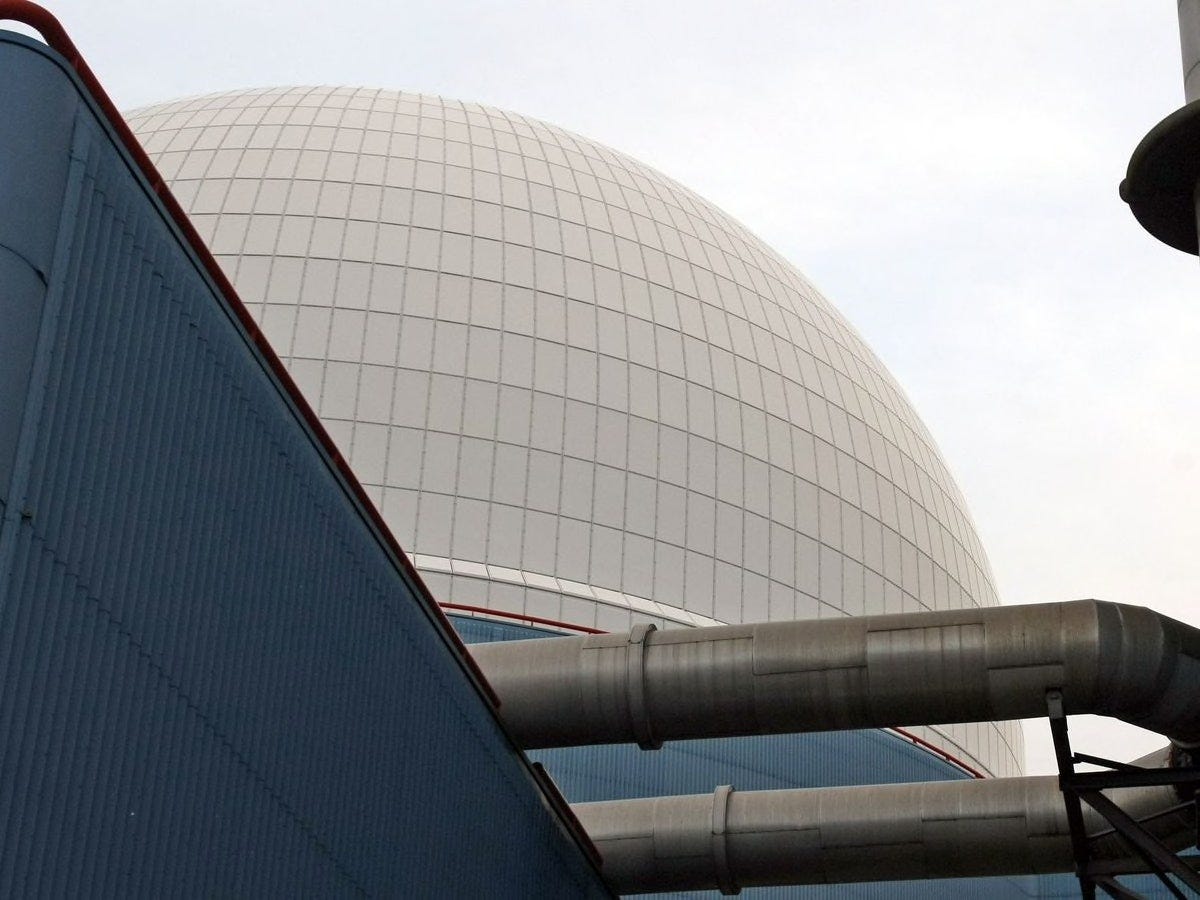
The steam generator is filled with separators and tubes. The tubes pump coolant through the generator to remove water from the steam. This prevents corrosion of the steam turbine blades and other parts of the generator. The nuclear energy industry uses the largest amount of water to cool the feedwater circling out of the steam generator and back to the pressure vessel. This amounts to approximately 1100 gallons (or 5000 litres) of water per megawatt hour of electricity produced. The coal industry used approximately 1005 gallons by comparison. Power stations are usually situated for this purpose near a river, lake or ocean.
So let’s look at how uranium heats the feedwater in the closed system. In the traditional system, uranium pellets are usually used to fill fuel rods. The more fuel rods the more power the reactor produces. The Uranium 235 isotopes are triggered (excited) by a starter neutron. As these isotopes are relatively unstable, with 92 protons and 143 neutrons, they are easily split. Each U-235 isotope splits and produces lighter elements and 2-3 more neutrons, which go on to collide with 2-3 more atoms. This chain reaction is similar to the gamma ray cascade seen when particles are hit by lightning-induced gamma rays. Then the moderator material, usually water, heavy water or graphite, is used to slow down the neutrons emitted from fission, in order that more collisions can occur, creating heat. The control rods, used to slow a reaction, can be made of neutron absorbing metals such as cadmium, hafnium or boron.
Whilst Nuclear power plants rely on fission to create heat, steam and turbine turning pressure in order to generate power, our simple electrical set up relies on just water current and corrosion resistant electrodes. Quite how efficient electrode/water current systems might be in comparison to nuclear power is difficult to assess.
It is clear, from the experiment at Stanford, that a considerable amount of battery power is achievable from the difference in charge of freshwater and seawater flowing over electrodes. The battery allows an element of control of how the power is stored and released, but the principle of seawater power generation is evident. It is possible that a continual feed of electrical current could be achieved from natural water flows.
Could an electrode system be developed alongside these other water technologies? Do we need turbines, steam, osmotic barries and gas to create energy or can our moving water create energy for us?
Next we are going to look at a drone creating energy at 100 metres off the ground, and how electricity transmission grids work.